The transduction of sensory stimuli uses direct or indirect activation of dedicated ion channels to generate a receptor potential. The mechanosensitive neurons of the fly’s Johnston’s organ are primary receptor cells that directly encode acoustic and gravitational information into a temporal pattern of action potentials. In contrast, hair cells and photoreceptors are secondary receptor cells using highly specialized ribbon synapses to drive excitation of the postsynaptic afferent neuron. Ribbon-type active zones are specialized for sustained transmitter release in response to graded changes of the receptor potential. We will study and compare cellular mechanisms of sensory encoding in the ears of flies and mice and in the retina.
Project |
Principal Investigator(s) |
Project Title |
A1 |
Göpfert |
Dissecting Drosophila auditory and gravitational stimulus transduction |
Project summary of A1
The fly’s ability to sense sound and gravity relies on force-gated ion channels transducing mechanical stimuli in Johnston’s organ neurons (JONs). Controversy has arisen over the molecular identities of these transduction channels: one model posits that different channels transduce sound and gravity, with the transient receptor potential (TRP) channel NOMPC (=TRPN1) being an integral component of the sound transducers. A second model assumes that NOMPC merely facilitates sound-transduction, and that the interdependent TRPs Nan and Iav mediate sound- and gravity-transduction. Testing these models requires information about (i) the TRP-dependence of individual JONs, (ii) the mechanisms of Nan and Iav activation, and (iii) searches for additional transduction-relevant channel proteins. Gathering this information is what this project is about: (i) the TRP-dependence of individual JONs will be imaged in vivo, (ii) the activation of Nan and Iav will be explored in vitro, and (iii) the sensory roles of candidate mechanotransducer components and channels will be analyzed, including Drosophila TMHS, Piezo, TMC, and Pain.
X close |
 |
A2 |
Moser |
Molecular physiology of hair cell transmitter release |
Project summary of A2
The ribbon synapses between cochlear inner hair cells (IHCs) and spiral ganglion neurons (SGNs) encode acoustic information with remarkable precision, reliability, and dynamics. Deciphering their molecular anatomy and physiology is critical in order to understand the mechanisms employed for accomplishing this challenging task. We have elucidated aspects of the spatial organization of Calcium channels and vesicles at the IHC active zone (AZ) and identified the ribbon protein complex, and the scaffold proteins bassoon, harmonin, and RIM2a in particular, as regulators of Calcium channel clustering and function. Our experimental and modeling data suggested a sophisticated stimulus-secretion coupling that builds on few Calcium channels in nanometer proximity of a given vesicle. There, one channel seems to dominate the Calcium concentration at the vesicular Calcium sensor, which likely involves a “molecular coupling” of the channel to the vesicular release site. Here, we plan to further test this “Calcium nanodomain” hypothesis of exocytosis control. We will employ the recently established paired pre- and postsynaptic recordings from single IHC ribbon synapses to biophysically test the “Calcium nanodomain” hypothesis. We aim to identify the protein(s) mediating the proposed molecular coupling at the IHC AZ. We hypothesize that otoferlin, RIMs and RIM-binding protein 2 (RBP2), and/or RBP2, bassoon and its vesicle-associated interaction partner mover, all of which are present at the IHC ribbon AZ, contribute to the proposed molecular coupling. We will first study potential effects of otoferlin, RIM3g and RBP2 on the function of CaV1.3 Calcium channels in HEK293/SK3 cells. The consequences of interfering with the RIM2, RIM3, RBP2, and mover genes for Calcium influx-exocytosis coupling and sound encoding will be investigated at various levels, from IHC and single synapse to systems function.
X close |
 |
A3 (E) |
Jahn / Urlaub |
Molecular mechanisms of synaptic vesicle docking |
Project summary of A3 (finished project)
Synaptic vesicles are the storage organelles for neurotransmitters that are accumulated in presynaptic nerve terminals. In the mammalian CNS, an active pool of synaptic vesicles is attached to specialized structures in the presynaptic plasma membrane, termed active zones. Despite major progress in the understanding of the active zone protein composition and both morphological and functional studies of mice lacking active zone proteins it is still not clear by which protein-protein interactions synaptic vesicles are attached. Furthermore, neurons involved in primary sensory signal transduction such as photoreceptor cells or inner hair cells of the organ of Corti contain a specialized synaptic structure, termed synaptic ribbon, to which synaptic vesicles are attached, but again the molecular nature of the link is not known.
The aims of the project were:
a) Which of the synaptic vesicle proteins are primarily involved in SV docking? In particular, are Rab effector complexes responsible for docking, and are SNARE interactions involved?
b) Which other proteins participate in the vesicle docking/fusion complex?
c) What is the protein composition of synaptic ribbons, and are the SV-ribbon connections formed by molecular interactions similar to those in CNS synapses? X close
|
 |
A4 (E) |
Reisinger / Urlaub |
Molecular machinery of the inner hair cell synapse |
Project summary of A4 (finished project)
In the 2015-2018 funding period, the groups of E. Reisinger and H. Urlaub joined their expertise and
achieved substantial progress towards a better understanding of otoferlin, a protein that is essential for exo-
cytosis from inner hair cells (IHCs), the sensory cells of the inner ear. Most importantly, we analyzed in detail the regulation of otoferlin by CaMKII delta (Meese et al., 2017) and the pathomechanism of the p.Ile515Thr mutation (Strenzke et al., 2016). Further work on identifying proteins of the synaptic vesicles of IHCs and on characterizing potential protein interaction partners of otoferlin is ongoing.. X close
|
 |
A5 (E) |
Rizzoli |
The molecular anatomy of synaptic vesicle recycling at the hair cell ribbon synapse |
Project summary of A5 (finished project)
The recycling of synaptic vesicles in inner hair cells (IHCs) is a fundamental step in hearing, which is still imperfectly understood. In the second funding period, we investigated this process by following two major directions of research: 1) To analyze the spatial organization and the copy numbers of molecular players in IHCs by different techniques, including a novel “comparative imaging” approach that we proposed. 2) To develop technologies for the analysis of the turnover of the molecules from IHCs, based on nanoscale secondary ion mass spectrometry (nanoSIMS). We have largely achieved these goals and have invested a large amount of work into developing and optimizing the technology required for the final analyses. First, we realized that the normal fixation and sample processing methods that are employed for imaging conventional cell cultures or tissues are poorly suitable for quantitative super-resolution analyses of sensitive tissues such as the cochlea, and especially the IHCs. We therefore undertook a major effort in testing all commercial fixative molecules available and found that the small dialdehyde glyoxal is an optimal fixative, which we adjusted for the needs of different tissues and of cells such as the IHCs (Richter et al., 2018, in collaboration with other PIs of the CRC 889, Moser and Vogl). Second, we developed the “comparative imaging” approach to a level where it is sufficient for quantitative analyses, and we are currently proceeding with the numeric characterization of proteins of the IHCs. Third, we developed a technology that combines fluorescence imaging with nanoSIMS and with advanced isotopic labeling, which can be used to study the turnover of organelles and molecule clusters in tissue with excellent resolution (Kabatas et al., 2015; Truckenbrodt et al., 2018a). Fourth, we have developed further imaging tools for the analysis of complex samples, ranging from click-able super-resolution probes (Vreja et al., 2015) to improved expansion microscopy with resolution of ~25 nm in multiple colour channels (thereby superior to all current commercial variants of super-resolution, including STED, STORM, or SIM; Truckenbrodt et al., 2018b).X close
|
 |
A6 |
Strenzke |
Hearing dysfunction due to pre- and postsynaptic deficits at the hair cell ribbon synapse |
Project summary of A6
Hearing relies on faithful sound encoding at the inner hair cell (IHC) ribbon synapse. Otoferlin is a synaptic protein that is specifically expressed in IHCs. Its absence abolishes exocytosis and causes deafness. However, the exact functions of otoferlin are yet unknown. In the first funding period, we performed a detailed analysis of sound encoding in a novel Otof missense mutant mouse (OtofI515T/I515T), which shows reduced otoferlin expression in IHCs and serves as a model for human temperature sensitive hearing loss. We found normal single auditory nerve (AN) fiber thresholds but abnormally strong adaptation to sustained sound stimulation and delayed recovery from adaptation as well as behavioral deficits, which are compatible with a function of otoferlin in vesicle replenishment. In addition, we dissected the molecular mechanisms of the sound coding deficit in Bassoon mutant mice which display reduced action potential rates in the AN due to perturbed ribbon anchorage and synaptic Ca2+ currents at the inner hair cell synapse1. We also established an experimental setup for recordings from the inferior colliculus2 and contributed auditory system physiology to several other CRC projects.
We now plan to analyze the consequences of AN action potential rate reductions with normal adaptation (hair-cell specific Bassoon knockout mice) and of pathological adaptation (OtofI515T/I515T) on auditory information processing in the brain. We will study sound-evoked responses of single neurons in the inferior colliculus and obtain behavioral measures of sound perception in Bassoon and OtofI515T/I515T mutants. We hypothesize that the reduced spike rates in Bassoon mutants will dramatically affect percepts that require coincident spiking such as amplitude modulation encoding, whereas the encoding of ongoing sounds and sound duration will be impaired in OtofI515T/I515T mutants. We also plan to analyze auditory function in PSD-95 knockout mice and spectrin mutants. We expect an AN spike generation deficit due to deficient AMPA and sodium channel receptor clustering. This work will also explore postsynaptic mechanisms that may contribute to AN fiber heterogeneity and adaptation, respectively. X close
|
 |
A7 |
Wichmann |
Activity modulated nanostructure of inner hair cell ribbon synapses |
Project summary of A7
The mechanisms underlying synaptic vesicle exocytosis at inner hair cell (IHC) ribbon synapses are subject of intense research. Interestingly, heterogeneous excitatory postsynaptic current (EPSC) shapes and amplitudes are observed which could originate from (i) synchronous release of two or more synaptic vesicles, (ii) larger vesicles derived from homotypic vesicle fusion prior to plasma membrane fusion, (iii) the simultaneous fusion of vesicles with each other and the plasma membrane, and/or (iv) high density of postsynaptic AMPA receptors, so that transmitter released from a single vesicle of normal size can elicit a large EPSC. Most likely, multivesicular fusion events would result in ultrastructurally visible tubular structures at the ribbon after stimulation. In our analysis using high K+ concentrations (50 mM, 15 min) for stimulation and subsequent serial 3D reconstructions and electron-tomography we did not detect such structures. Instead, we found an enrichment of larger vesicles after stimulation at the membrane-distal part of IHC ribbons.
In order to refine our analysis of the ultrastructure of functional active zone states we plan to establish optogenetics in IHCs in combination with transmission electron microscopy (EM). In this manner, after expression of Channelrhodopsin-2 constructs, even very short depolarizing stimuli (milliseconds) can be applied precisely and non-invasively using light. In combination with high-pressure freezing within milliseconds after stimulation, we will capture the synaptic ultrastructure of a given functional state in a near native condition.
Different stimulation protocols will be applied and analyzed. We will test short (10 ms) and long stimuli (200 ms) combined with various recovery durations and analyze the number of membrane proximal or tethered and ribbon associated vesicles and determine their size using electron-tomography and serial 3D reconstructions. The time-point of appearance and clearance of the population of large vesicles described above will also be in our focus.
Moreover, our interest lies on the molecules involved in release, especially on how the tethering of synaptic vesicles to the ribbon or the membrane is organized. Quite likely, the tethering of synaptic vesicles to the ribbon is mediated by other molecules than those mediating tethering to the active zone membrane that likely prepares a vesicle for subsequent fusion. We will characterize tether classes present at IHC ribbons, their position and length in high-pressure frozen wild type and mutant IHCs. We will investigate otoferlin mutants, RIM1/2 and Myosin VI knockout mice, and Piccolo/Piccolino gene-trap knockout rats for the role of the affected proteins in tether formation.
This way we expect to provide a major contribution to the so far unsolved mechanism of vesicle release at IHC ribbon synapses and the proteins involved. X close
|
 |
A8 (E) |
Milosevic / Moser |
Molecular physiology of synaptic vesicle recycling at the hair cell ribbon synapse |
Project summary of A8
Efficient synaptic vesicle recycling is of particular importance for the auditory inner hair cells (IHCs) whose synaptic vesicle (SV) release rates are exceptionally high. Yet, relatively little is known about the mechanisms of SV recycling at IHC ribbon synapses, and the relevance of hair cell endocytosis for synaptic sound encoding is not well understood.
In our previous work, we have studied the function of endophilin A1-A3 at conventional synapses. Here, we have started to dissect the function of major endocytic proteins, endophilin, dynamin, adaptor protein 2 (AP-2) and adaptor protein 180 (AP180) at the IHC synapse. We have employed multi-disciplinary approach and various techniques, ranging from mRNA analysis, immunohistochemistry, confocal and STimulated Emission Depletion (STED) microscopy, electron microscopy (EM) and tomography as well as electrophysiological recordings from IHCs and the auditory system in relevant mouse mutants. We found that all aforementioned proteins are expressed in the IHCs, and that the SV release in IHCs is tightly balanced by robust membrane retrieval and SV reformation. Specifically, we showed together with our collaborators that endophilin, AP-2 and AP-180 have several functions along the SV cycle, and their absence appears to be rate-limiting for the clathrin-dependent reformation of SVs from the endosome-like vacuoles. In particular, the absence of endophilin modulated presynaptic calcium channels and resulted in deficient SV recruitment to the ribbon and/or impaired release site clearance at the IHC synapse.
Taken together, our studies on endocytic proteins at the IHC ribbon synapse revealed tight and balanced coupling between exocytosis and endocytosis, and demonstrated the unexpected robustness of SV recycling even in the absence of some of the key endocytic proteins. X close |
 |
A9 |
Schmidt |
Molecular characterization of Piezo2-dependent mechanosensation |
Project summary of A9
Piezo2 ion channels are major mechanotransducers for vertebrate light touch and proprioception. Our recent work has revealed that a comprehensive protein network, i.e. interactome, regulates Piezo2 ion channel function in peripheral sensory neurons. Our current research efforts aim at investigating (i) how Piezo2 ion channels are regulated throughout physiological development and aging in mice, (ii) which molecular mechanism are involved, and (iii) how this might affect mouse behaviors related to light touch and pain. We expect results derived from this project to reveal novel insights into physiological plasticity of Piezo2 – with significant implications for understanding and modulating dysfunctional somatosensory mechanosensitivity in vertebrates.
X close |
 |
A10 (N) |
Clemens |
Biophysical and molecular mechanisms of mean and variance adaptation in Drosophila auditory receptor neurons |
Project summary of A10
Adaptation is ubiquitous in the nervous system and supports efficient sensory coding. However, the mechanisms and molecules underlying adaptation are often unknown. Drosophila is an ideal model system for analyzing the mechanisms of adaptation since powerful genetic tools allow one to manipulate and monitor the activity of the nervous system down to the function of individual proteins.
The fly’s antenna houses a diverse population of mechanosensory neurons – so-called Johnston’s organ neurons (JONs). Subsets of these neurons are sensitive to fast antennal vibrations induced by sound – most
notably to the song produced by males during courtship. These JONs thus serve as the auditory receptor neurons. We have recently described the interaction between two distinct forms of adaptation in JON (Clemens et al., 2018) adaptation to the mean of the mechanical stimulus to correct for the baseline position of the mechanical receiver; and adaptation to the variance of the mechanical stimulus – equivalent to the sound intensity – to maintain sound sensitivity across a wide dynamic range. By evaluating receiver motion, generator currents, and spiking rates, we showed that mean adaptation first arises at the level of mechanotransduction, while variance adaptation arises downstream, but before spike generation, and that both forms of adaptation are independent of each other.
Here, we aim to build up on this work and identify the molecular and biophysical basis of mean and variance adaptation in JONs. Several classes of molecules are expressed in JONs that are candidates for implementing the two forms of adaptation, e.g., potassium channels or molecules that are part of signaling cascades supporting adaptation in other sensory neurons, e.g. photoreceptors. We will combine genetic knockdown or
knockout of candidate molecules with electrophysiological and optical read-outs of receiver motion, generator currents, and spiking rates to identify how and at which stage these molecules affect adaptation and neural coding in general. A computational model of the complete transformation from mechanical stimulus to spiking rate developed previously (JC, unpublished data) will serve 1) as a tool to quantitatively describe changes in coding properties upon manipulation of individual molecule types and 2) as a scaffold to integrate our experimental findings and derive novel hypotheses about how different forms of adaptation are imple-
mented to efficiently present sensory information.
X close |
 |
A11 (N) |
Sakata / Preobraschenski |
Structural and functional analysis of the multi-C2-domain protein otoferlin |
Project summary of A11
Otoferlin plays a key role in neurotransmitter release in inner hair cells. It is a member of the multi C2 domain protein family referred to as ferlins and contains six or seven C2 domains, a coiled-coil domain, a ferlin specific FerB-motif and a single transmembrane domain at the C-terminus. Despite advances in understanding the function of otoferlin in inner hair cells, it remains unclear how the multi C2 domains operate in synaptic vesicle exocytosis. This is primarily owed to the challenges encountered when trying to purify functional full-length otoferlin in sufficient amounts to gain structural insights of otoferlin, which are pivotal for our understanding of its molecular mechanism. Capitalizing on our recent breakthrough in purification of full-length otoferlin, we plan to address its biochemical and biophysical properties and to determine its three-dimensional structure. We will apply biochemical and biophysical assays to investigate functional and structural features of otoferlin in the presence or absence of cofactors (Ca2+, lipids, interacting proteins). Based on these experiments, we will optimize purification conditions and determine the otoferlin structure by cryo-EM single particle analysis. We expect this research to yield novel insights into the molecular mechanism of otoferlin-involved exocytosis.
X close |
 |
Following encoding and afferent transmission of sensory information the sensory system accomplishes important tasks such as adaptation, improvement of spike timing, and contrast enhancement. The projects of area B and C combine multidisciplinary expertise exploring principles of central sensory processing. The B area combines expertise on the cellular mechanisms underlying mechanisms of plasticity using dedicated model systems. Whereas neuronal plasticity and sensory processing are sometimes regarded as separated neuroscientific fields, this CRC aims at integrating these two areas of research. We strongly believe that investigating phenomena of neuronal plasticity, be it learning or sensory adaptation, put explicitly into the context of sensory processing, will be enormously beneficial for a comprehensive understanding of how sensory stimuli are computed by nervous systems. The projects B1 – B3 investigate cellular mechanisms of synaptic plasticity in the context of sensory processing, focusing on well established neuronal preparations. The projects B4 and B5 go a step further by investigating sensory processing on whole-animal models, with project B4 focusing on the olfactory system of Drosophila, and B5 on the visual system of mice.
Project |
Principal Investigator(s) |
Project Title |
B1 |
Rhee / Brose |
Genetic dissection of synaptic vesicle priming and Ca2+ sensing in rod bipolar cell ribbon synapses of the mouse retina |
Project summary of B1
Vesicular release of neurotransmitters at presynaptic active zones (AZs) is extremely fast and tightly regulated by Ca2+ ions. The two main reasons for the speed of excitation-secretion coupling are that synapses maintain a pool of release-ready or primed synaptic vesicles (SV) at the AZ, and employ a vesicular Ca2+ sensor that boosts the SV fusion rate by up to 1 million fold upon an increase in the cytosolic Ca2+ concentration. Retinal ribbon synapses are unique in this regard because they exhibit 5-10 times higher priming rates than conventional synapses, and release transmitters in response to graded potentials over a broad dynamic range. The molecul ar basis of these unique features of ribbon synapses is largely unknown. We propose to study SV priming and Ca2+ sensing in mouse retinal ribbon synapses, and the role of these processes in determining the unique functional features of ribbon synapses. We will use the rod bipolar cell (RBC) to AII amacrine cell connection as our model, and will focus our efforts on mice lacking the core protein components of the presynaptic SV priming (Munc13s, CAPSs) and Ca2+ sensing machinery (Synaptotagmins, Complexins). We will analyse synaptic transmission at these synapses in wild-type and KO mutant mice, and will complement these studies with analyses of the ultrastructure of RBC synapses. We expect that our studies will reveal a key role of SV priming and Ca2+ sensing proteins in determining the unique properties of retinal ribbon synapses.
X close |
 |
B2 (E) |
Dresbach |
Role of the presynaptic protein Mover at auditory brain stem synapses |
Project summary of B2
Temporal precision and use-dependent plasticity are hallmarks of synaptic transmission in the auditory pathway. During the first funding period, the project revealed that the presynaptic protein Mover occurs at inner hair cell ribbon synapses of the cochlea, at a subset of excitatory synapses - called endbulbs of Held – contacting bushy cells in the cochlear nucleus, and at the majority of inhibitory synapses in the cochlear nucleus. Biochemical characterization revealed that Mover is a dimerizing phospho-protein of synaptic vesicles. A collaborative study showed that knockdown of Mover at the calyx of Held increased presynaptic release probability and short-term depression. In mice with impaired inner hair cell function, Mover was decreased at endbulbs of Held, raising the possibility that Mover is an activity-dependent regulator of presynaptic strength. We extended these studies to i) determine the role of Mover at excitatory and inhibitory synapses in the cochlear nucleus, ii) characterize its contribution to homeostatic plasticity in hearing impaired mice, and iii) determine the underlying molecular mechanisms by analyzing how the expression and localization of Mover are regulated. Our primary tool was a floxed Mover mouse line that we created in the first part of the project. This allowed us to use specific Cre mice (including Synapsin1:Cre, VGlut1:Cre, VGAT:Cre) to delete Mover from distinct subsets of neurons while leaving the gene intact in inner hair cells. As a major readout we patched clamp bushy cells in the cochlear nucleus and stimulated their presynaptic inputs. To test the contribution of Mover to homeostatic plasticity, we analyzed bushy cell synapses in hearing impaired mice. Using cultured neurons we tested how the expression and localization of Mover are regulated during homeostatic plasticity. Finally, in CRC collaborations, we used cell-type specific deletions of Mover to determine its role for inner hair cell function, and for circuit activity in the auditory brainstem. The project elucidated the role of Mover at auditory synapses.
X close |
 |
B3 |
Schlüter |
Cellular and Molecular Mechanisms of (Primary) Visual Cortex Network Refinement |
Project summary of B3
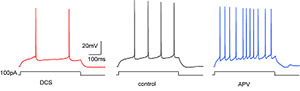 Cortical networks undergo experience-dependent modifications during critical periods, time windows of elevated cortical plasticity, which are necessary to shape network function for optimized sensory signal processing. Sensory deprivation during these periods irreversibly impairs sensory perception later in life. The PSD-95-related protein family constitute signalling scaffolds, which were shown to regulate signalling events during long-term synaptic plasticity. Furthermore, PSD-95 has been shown to regulate excitatory synapse maturation. In this project, we will test the role of these paralogs in visual cortex maturation. Using gene target mice and viral vector-mediated gene transfer, we will test the role in synaptic maturation with ex vivo primary visual cortex V1 slice preparations and in collaboration with B5 cortical critical period plasticity.
X close |
 |
B4 |
Fiala |
Neuronal Mechanisms of Olfactory Learning in Drosophila: Thermogenetic and Optophysiological Approaches |
Project summary of B4
Despite our increasing knowledge about how sensory stimuli are encoded by neuronal circuits and how these stimuli guide behaviour, much less is known about how internal states are integrated by neuronal circuits. The metabolic hunger/satiety state of the animal, and the past experience of available food of high or low caloric value, represent parameters that strongly influence innate behaviour and learning. We use the fruit fly Drosophila to analyze structural neuronal plasticity that is dependent on the long-term experience of the caloric value of food. In particular, we aim at functionally dissecting how the experience of food uptake with high or low caloric value structurally and functionally shapes those neuronal circuits in the mushroom body of the Drosophila brain that organizes olfactory behaviour and learning. We have found that a small subset of dopaminergic neurons innervating the mushroom body influence food-uptake, and undergo structural and functional modifications dependent on the animal’s past experience of the caloric food value. This will serve as an experimental paradigm to understand how neuronal circuits orchestrating innate and learned behaviour are modified in dependence of internal states.
X close |
 |
B5 |
Löwel |
The role of the postsynaptic signaling scaffold PSD-95 for visual cortical function and plasticity |
Project summary of B5
Sensory-cortical development peaks during critical periods, time windows of heightened synaptic plasticity that allow experience-dependent fine-tuning of both structural and functional properties of cortical neurons, a process essential for proper stabilization and optimization of cortical processing architectures. PSD-95 and PSD-93 are signaling scaffolds of the postsynaptic density of excitatory synapses and we could recently show (with B03) that these proteins have opposing functions for experience-dependent optimization of visual cortical circuits: while PSD-95 is necessary for maturation of silent synapses and closure of the critical period for ocular dominance plasticity, PSD-93 prevents precocious synaptic maturation. In this project, and in close collaboration with B3, we will further test the role of PSD-95 and PSD-93 for visual cortical function and plasticity: we will record from single neurons while mice learn a visual discrimination task, use in vivo 2-photon imaging to record Ca2+-transients during visual stimulation, and study the role of the signalling scaffolds for recovery from amblyopia.
X close |
 |
B6 (E) |
Dean |
The role of synaptotagmins in olfactory processing |
Project summary of B6 (finished project)
Regulation of both neurotransmitter and neuropeptide release is important for the reliable detection and discrimination of different odors. Different synaptotagmin isoforms - which regulate neurotransmitter and neuropeptide release - localize to different layers of the olfactory system at the mRNA level, suggesting a specialization of regulated exocytosis at different levels of olfactory processing. In this project we aimed to localize the different syt isoforms at the protein level in the olfactory bulb, and to determine the function of these syt isoforms in olfactory processing and odor discrimination.
Syt isoforms that promote vesicular fusion sense calcium through their C2 domains, and these syts have different ranges of calcium sensitivity. Syt-1 is present on synaptic vesicle membranes and is essential for fast synaptic vesicle exocytosis. Syt-4 is localized to neurotrophin-containing vesicles, where it inhibits BDNF release to affect synaptic strength in the hippocampus. Syt-7 is involved in lysosomal fusion. Syt-10 is reported to regulate release of IGF-1 in the olfactory bulb. However, the function of most syt isoforms is still unknown.
Synaptotagmins are highly conserved and most are expressed in brain. Our recently published pHluorin screen of syt isoforms in neurons indicates that many syts may regulate neuropeptide release. Interestingly, these syts are differentially expressed in specific brain areas and sensory systems, suggesting a specialization of synaptic function and plasticity.
X close |
 |
B7 (E) |
Willig |
Nanoscopy of visual cortex plasticity |
Project summary of B7
The most basic functional units for sensory processing in the brain are synapses formed by a presynaptic and a postsynaptic part of two neurons. Although the overall morphology of the dendritic arborisation of adult neurons is considered to be rather stable, postsynaptic spines change in shape and size, appear and disappear. These morphological changes relate to variations of synaptic strength and formation or elimination of synapses, respectively. There is evidence that these synaptic changes are experience-dependent and in their sum influence the function of the neuron and the entire nerve cell network. The study of these morphological changes in response to sensory stimulation is only possible when the whole neuronal circuit is intact, which is only the case in the living organism. In the mouse primary visual cortex, for example, spine plasticity can be easily evoked by visual deprivation or retinal lesion. These structural changes can be visualized with in vivo two-photon microscopy with a resolution of roughly 300-500 nm. However, important morphological details, such as the precise shape and size of postsynaptic spines and presynaptic boutons, are not accessible because of the diffraction-limited resolution. We have recently implemented in vivo STED microscopy, a novel light microscopy technique with sub-diffraction limited resolution, to resolve structural changes in unprecedented detail. We have imaged neurons of the visual cortex of an anesthetised mouse at a resolution of 60 nm and showed that postsynaptic spine necks and presynaptic axons are below 100 nm in size. Imaging the actin cytoskeleton in dendritic arborisations at a resolution of 50 - 70 nm opened the possibility to record sub-cellular structures in vivo. Of all the nanoscopy or superresolution techniques, STED microscopy stands out for its fast recording speed, sectioning capability and the potential to image tens of µm deep into living tissue.
In this project, we will use STED microscopy to explore spine plasticity in layer 1 of the primary visual cortex (V1) in much greater detail than previously possible. We will visualize structural changes of the spines during visual cortex plasticity, which cannot be captured with two-photon microscopy. Specifically, we will characterize morphological changes of the spine head and neck, which influence synaptic signalling, and classify spines according to standard electron microscopy categories into ‘stubby’, ‘mushroom’, ‘thin’ or ‘filopodia’. We will visualize spine plasticity in layer 1 of visually deprived mice and in mice which are exposed to different light stimuli after visual deprivation. Evidence for changes of spine morphology, which are tightly correlated with activity-dependent changes of the network, have previously been shown in layer 1 of V1 using two-photon microscopy. For visual deprivation we will use a brief dark exposure (14 days), which has recently been shown to restore the vanished ocular dominance plasticity in V1 of adult and aging mice by the Löwel group (B5). Furthermore, to extend our initial work on visualizing actin, the most abundant cytoskeletal protein in spines and involved in spine plasticity, we will study actin-binding proteins and image their spatial distribution in dendritic spines in vivo. For this purpose, we will also advance in vivo STED microscopy towards the use of red-fluorescent proteins for deeper penetration and improved photostability.
X close |
 |
B8 (N) |
Vogl / Enderlein |
Illuminating the morphological and functional plasticity of cochlear inner hair cell ribbon synapses |
Project summary of B8
Hearing relies on the temporally precise and ultrafast synaptic sound encoding between cochlear inner hair cells (IHCs) and postsynaptic spiral ganglion neurons. These synapses are characterized by the presence of a presynaptic electron-dense body – the so-called 'synaptic ribbon'. Ribbons contribute to the clustering of voltage-gated Ca2+ channels at the release site and tether a supply of synaptic vesicles, thereby ensuring rapid vesicular replenishment even during periods of intense and prolonged stimulation. To date, little is known about the morphological plasticity, mobility and turnover rate of these seemingly rigid dense bodies.
Moreover, basic features of IHC ribbon function, such as its definitive role in vesicular release and replenishment as well as its capacity for presynaptic plasticity – in particular during periods of ongoing or recurrent activity – remain elusive. To address these issues, we employ a multidisciplinary experimental approach, combining rapid and long-term live-cell imaging with different optogenetic stimulation paradigms in vitro. The collected data will provide novel insights into the presynaptic dynamics and function of auditory ribbon synapses and shed light on the adaptive capacity of its unconventional release machinery.
X close |
 |
B9 (N) |
Pangršič Vilfan |
Synaptic mechanisms of noise-induced hearing loss |
Project summary of B9
Noise-induced hearing loss (NIHL) is the most frequent occupational disease in Germany. Milder noise exposure that used to be considered as “safe” may result in so called “hidden hearing loss”, as observed in various animal models. This only temporary attenuation in hearing sensitivity involves irreversible loss of a large fraction of inner hair cell synapses. Such “hidden hearing loss” is not routinely being tested for in clinics. But, it has been indicated to also contribute to NIHL in humans, where it may reduce the ability to understand speech in noisy backgrounds. It may also accelerate or worsen age-related hearing loss, the most common form of sensorineural hearing impairment in today’s society. This project aims at investigating adaptive and/or pathological processes activated in the inner ear upon noise trauma. We will utilize mutant mouse models, optogenetic tools and a number of in vitro and systems physiology approaches to unravel the mechanism of noise-induced changes in the organ of Corti. Finally, we will aim at ameliorating noise-induced damage by application of drug(s) that may in the future be used for causative treatment of such hearing impairment.
X close |
 |
Projects in area C join to explore the cellular and network mechanisms underlying integration and representation of sensory information as well as by bottom-up and top-down modulation of sensory processing in the central nervous system. To do so the projects will use a large array of experimental and computational approaches to the central nervous system as “simple” as in Drosophila and as “complex” as that of primates.
Project |
Principal Investigator(s) |
Project Title |
C1 |
Gollisch |
Circuit mechanisms underlying signal processing in the mammalian retina during saccades |
Project summary of C1
Natural vision is structured by brief periods of fixation, separated by rapid shifts in the direction of gaze, known as saccades. These visual dynamics shape the signals that impinge on the retina at the back of the eyeball. Saccades thereby strongly affect how visual information is processed by the retinal network and how it is encoded into spike patterns of retinal ganglion cells, the output neurons of the retina. The goal of this project is to elucidate the cellular and synaptic features in the neural circuit of the retina relevant for the processing of visual signals in the presence of saccades. Our recent work has revealed that ganglion cells of mouse retina display diverse types of saccade-elicited responses. In particular, some cells show unexpected dependencies on the transition from pre- to post-saccadic images: they respond selectively, for example, when the image content inside the receptive field either does or does not change across the saccade. We therefore aim at deciphering the neural mechanisms in the retina that underlie the diverse response types and that generate the surprising selectivity for certain types of image transitions. Our hypothesis is that the saccadic image transition triggers specific inhibitory interactions, which lead to signal processing characteristics that go beyond the classical receptive field model of retinal ganglion cells and that are typically not observed with standard, stationary stimulation. Furthermore, adaptation mechanisms, in particular synaptic depression, are likely to contribute to shaping responses across saccades.
To assess the contributions of different circuit mechanisms in shaping the saccade-elicited responses, we will combine several experimental and computational approaches. We will use extracellular multielectrode-array recordings to thoroughly test how the response properties of retinal ganglion cells are affected by various modifications of the saccadic stimulation. We will use intracellular patch-clamp recordings to characterize the cells’ excitatory and inhibitory inputs in the presence of saccadic stimulation. We will use pharmacological blockers to disentangle the contributions of different signalling pathways and different types of inhibitory interactions. And finally, we will use computational modelling and comparison with responses to saccades in natural stimuli to probe whether the identified mechanisms are sufficient to explain the diverse response types. The project will thereby contribute to our understanding of visual processing in the presence of natural visual dynamics. Moreover, given the prominent role of inhibition in this processing, as demonstrated by our preliminary data, the project provides an opportunity to study the function of the intricate and still poorly understood network of inhibitory amacrine cells in the inner retina in the context of a natural visual task. close |
 |
C2 (E) |
Göpfert / Fiala |
Multimodal signal integration in the Drosophila Brain |
Project summary of C2 (finished project)
Drosophila is widely used to investigate mechanisms of sensory stimulus processing at the level of sensory signal transduction in sensory organs, at the level of neuronal circuits processing sensory stimuli, and at the level of behavior. In most studies one sensory modality is investigated for how these stimuli contribute to inducing and modifying behavior. In only very rare cases, however, the integration of two or more stimulus modalities has been considered. We reasoned that signal integration across modalities represents a crucial feature of any brain functioning, and that this missing link needs to be overcome. The Fiala lab focuses on the sense of olfaction, the Göpfert lab on mechanosensation. Both sensory modalities are used by the animal in various contexts, e.g., for courtship and mating decision or for tracking odor plumes. In project C2 we proposed to analyze neuronal signal integration of mechanosensory and olfactory cues in the Drosophila brain.
X close |
 |
C3 (E) |
Staiger / Lampl |
Integration of afferent sensory and cortical input by identified neurons of the rodent somatosensory cortex |
Project summary of C3 (finished project)
Processing of tactile information emanating from the mechanoreceptors of the whiskers is mediated by at least 3 parallel pathways, which ascend through subcortical processing stations to the somatosensory (barrel) cortex where they are believed to target different layers and areas. It is largely unclear, however, if they also differentially target specific types of cells and in turn if these cells further receive different types of local cortical inputs. Our main goal is therefore to determine, at the level of identified layer IV cortical neurons, the relationship between sensory response properties (such as receptive field size, directional selectivity and sensory adaptation or network dynamics) and their functional and structural innervation (from specific thalamic pathways as well as identified local cortical inputs). Toward this aim, we combined specific stimulation of facial whiskers, multiple intra- and extracellular electrophysiological recordings as well as genetic anterograde and retrograde tracing methods and performed both, in vivo and in vitro (thalamo-cortical slice) experiments in the rodent whisker-to-barrel pathway(s). Beyond clarifying cellular, synaptic and circuit mechanisms of tactile information processing, these experiments will be further useful to ultimately achieve specific optogenetic manipulation of individual cells and circuits in behaving animals. By either stimulating or inhibiting them, we can probe their contribution to sensory guided behavior.
X close |
 |
C4 |
Treue |
The pharmacology of attention in fronto-parietal sensory information processing |
Project summary of C4
There is accumulating evidence that the cholinergic system may play an important role in the attentional enhancements of sensory responses in the visual cortex. We began to investigate this hypothesis in the first funding period in extrastriate area MT, where neurons are strongly tuned for the direction of visual motion in their receptive field and firing rates are reliably enhanced by the allocation of spatial and feature-based attention. We recorded from single cells in an awake, behaving rhesus monkey during a spatial attention task in which a moving stimulus in the receptive field was attended or unattended. During recordings, we used pressure injections to pharmacologically manipulate cholinergic receptors in the direct vicinity of the electrode. The pattern of attentional modulation during injection blocks was compared to baseline blocks recorded before injection. Spatial attention significantly enhanced firing rates by about 10-20% while local application of the muscarinic antagonist scopolamine significantly lowered firing rates by about the same amount. However, the two effects seem to be independent, with no indication of a stronger scopolamine-related decrease in firing rate when the animal was attending to a stimulus in the receptive field. Although previous work in macaque V1 has shown a strong contribution of the muscarinic receptor subtype to attentional enhancement of firing rates, our results suggest that this is not the case in MT.
In the three subprojects of the second funding period we will complete the experiments from the first funding period and expand our study:
- We will complement the data we have collected in the current funding period (one animal, muscarinic antagonist scopolamine) by collecting data from a second animal and by applying the nicotinic antagonist mecamylamine and the agonist acetylcholine. If none of these substances show an influence on the attentional modulation we will expand our investigation to include other neurotransmitter systems.
- We will expand the scope of our behavioral task to include feature-based attention to compare the neuropharmacological effects on this attentional subtype with the effects we observed for spatial attention.
- We will continue our experiments from the current funding period aimed at understanding the role of the Frontal Eye Field (FEF) in the spatial specificity of attentional modulation in area MT.
X close
|
 |
C5 |
Gail |
Sensory cue integration in the fronto-parietal sensorimotor network |
Project summary of C5
Multisensory integration is a key function for spatial cognition and motor planning. This project investigates sensory cue integration in the sensorimotor network of primates. The project has a special focus on the context-dependency of visuospatial cue integration and the role of functional interactions between parietal lobe and frontal lobe sensorimotor areas during reach planning. In the first funding period we addressed the open questions (a) whether spatial representations of visually instructed reach goals in the parietal cortex of rhesus macaques can be observed in situations when the final reach goal is not yet finally decided upon but rather remains ambiguous do to an unresolved behavioral task rule, and (b) whether our previous hypothesis that respective spatial representations in the parietal cortex are contingent upon functional input from frontal lobe areas can be confirmed by directly demonstrating such functional connectivity. As a result of the first funding period we could show that neurons in the parietal and premotor cortex of rhesus monkeys encode preliminary stages of cue integration when a visuospatial cue has to be integrated with an ambiguous non-spatial visual cue. Also, we found frontal-to-parietal functional neural interactions during tasks which require integration of two such cues.
We now intend to further analyze the functional role of the observed fronto-parietal neural interactions during multisensory integration. We are expanding the scope to behavioral task which require the integration of sensory cues from different sensory modalities, namely visual and somatosensory cues, since both sensory domains are highly relevant for motor planning. Specifically, we are testing under which behavioral conditions the observed frontal-to-parietal functional interactions are effective prior to the onset of a behavioral response. Specifying the conditions under which frontal cortex areas impose a functional input to parietal space encoding will help to narrow down the functional role of these interactions and to better understand the mutual roles of parietal and frontal lobe sensorimotor processing.
X close |
 |
C6 |
Wolf |
Drift, matching, and stabilization of visual cortical representations |
Project summary of C6
How are cortical representations of converging sensory streams matched and stabilized under the influence of livelong plasticity? This question is fundamental to the function of cortical sensory representations. Until recently it was widely believed that cortical representations are largely fixed by the closure of juvenile periods of developmental plasticity. This view has been challenged by observations of ongoing reorganization of cortical representations in various adult cortical systems such as barrel cortex, and rodent and primate motor cortex. In the first funding period of CRC 889 in collaboration with Löwel (B5), we developed a dynamical systems theory for the stabilization of mouse visual cortical organization. This theory explains the spatially disorganized arrangement of orientation preferences in the mouse visual cortex by the influence of dense local inhibition on the coordinated plasticity of cortical neurons. The current project develops a framework for the characterization and analysis of such dynamically labile orientation representations and uses this theory for dissecting the impact of genetic depletion of the postsynaptic density protein PSD-95 on the structure and dynamics of orientation representations in the primary visual cortex. We will collaborate with Löwel (B5) & Schlüter (B3) to quantitatively characterize and mathematically model the distribution of receptive field drift, matching and stability in normal visual cortex and in animals with genetic PSD-95 depletion (“V1 Alliance”).
X close |
 |
C7 |
Staiger |
The role of cortical GABAergic interneurons in pathway-specific control of sensory information processing |
Project summary of C7
A strong notion in sensory systems neuroscience states that inhibitory interneurons shape sensory perception by feedforward and feedback inhibition of excitatory principal neurons. However, several publications have recently shown that sensory circuit mechanisms exist in vitro and in vivo that are characterized by disinhibitory effects mediated by the connections of a specific type of cortical GABAergic interneuron, i.e. the VIP-expressing bipolar/bitufted cell, onto distinct classes of other inhibitory interneurons. These pathways, which seem to be a canonical motif in all cortical areas, were previously predicted by us based on correlated light and electron microscopy and in vivo electrophysiology. With specific cre-driver lines and optogenetics, we now plan to study the functional connectivity of VIP neurons, in terms of their activation by the different ascending thalamic pathways that convey sensory information and context for conscious perception to the cortical circuits. We are working on the following questions: (i) Which pathways do innervate VIP-expressing cells (but also PV-expressing basket cells and SOM-expressing Martinotti cells in comparison)? In this in vivo-stereotactic viral vector injection project, a floxed rabies virus is injected in small quantities to label the cre-expressing inhibitory interneurons of the supragranular, granular and infragranular compartment, respectively. With that approach we will identify all presynaptic thalamic input neurons of the three postsynaptic interneuron types, together with the contextual inputs coming from specific layers of cortical areas and a multitude of subcortical, non-thalamic nuclei. (ii) Which thalamic pathways do activate VIP-expressing irregular- or burst-spiking cells (but also PV-expressing fast-spiking basket cells and SOM-expressing adapting Martinotti cells in comparison)? In an approach combining in vivo-stereotactic viral vector injections (AAV-based channelrhodopsins into the different thalamic subnuclei that were identified as presynaptic sources of inputs to the different types of interneurons in the different layers of the barrel cortex in the previous project) with in vitro slice work, we will investigate, if and to what extent a pathway-specific innervation does exist. Finally, by using subcellular channelrhodopsin-assisted circuit mapping we can analyze their somatodendritic location on the patched target cell. (iii) What are the functional consequences of activation or inhibition of VIP interneurons on the canonical cortical microcircuit? Here, we plan to test our hypothesis that a prominent disinhibitory circuit involves layer (L) III/IV VIP cells that project onto LIV PV basket cells. Therefore, we will use electrical stimulation of a thalamo-cortical slice that is submerged in activity-promoting ACSF that allows producing stimulus-evoked activity in most of the cortical cells. The slice comes from a transgenic VIPcre/PV-eGFP mouse into which a floxed ChR2 or NpH (and RFP) expressing virus was injected into the barrel cortex to transduce interneurons in all layers of at least one column. We will do the following recordings: (a) from VIP neurons in several layers to prove that they are excited by blue laser light when transduced with ChR2 or inhibited by yellow laser light when transduced with NpH; (b), from LIV-PV interneurons to check whether they fire more/less during stimulus-evoked UP-/DOWN-states and how this is modulated by activating or inhibiting VIP neurons; (c) from LIV or LII/III principal neurons to see whether the manipulation of the putative VIP-to-PV disinhibitory circuit changes the feeding forward of sensory information from LIV to LII/III along the canonical microcircuit. These experiments will greatly improve our understanding of disinhibitory mechanisms contributing to sensory information processing.
X close |
 |
C8 |
Silies |
The molecular, cellular and network basis for direction-selective inhibition in early visual processing |
Project summary of C8
A hallmark of detecting visual motion is the ability to respond to signals moving in different directions, rather than static contrast changes. Neurons that fulfill this criterion, so-called direction-selective (DS) cells, have been identified in several organisms. Understanding the mechanisms underlying direction-selectivity at a circuit and cellular level will reveal how defined neural computations are implemented in the nervous system.
In the Drosophila visual system, direction-selectivity is thought to first arise in T4 and T5 cells in the lobula complex, the columnar inputs to wide-field cells that integrate motion across space. Own unpublished data show that DS signatures already exist several synapses upstream in peripheral visual processing in a first order interneuron, whose DS-tuning is present in a signal component that likely arises through lateral inhibition. Here, we plan to isolate the mechanisms underlying directed lateral inhibition in early visual processing. Pharmacological manipulations will first determine the extent by which inhibitory GABAergic inputs shape DS inhibition. We are then going to use cell type specific genetic manipulations to identify the subcellular machinery and the circuit architecture that are required to establish lateral inhibition in first order interneurons. Finally, the extent by which these early DS features are utilized by downstream circuits to achieve strong DS tuning in neurons such as T4 and T5 will be modeled in collaboration within the consortium, and tested experimentally.
X close |
 |
C9 |
Scherberger |
Visual and tactile signal processing for high-order object recognition |
Project summary of C9
How does our brain perceive objects that we manipulate or touch, and how does it represent object information relevant for its manipulation? Are grasping actions planned differently for objects that are perceived visually or by touch? Answers to these questions are at the heart of understanding better how sensory object information is processed in the brain for the planning and execution of grasping movements, and for understanding sensorimotor transformation in general. To explore these questions, we will perform electrophysiological recording and temporal inactivation experiments in behaving non-human primates while they are performing grasping or object discrimination actions. We will investigate to what extent high-order sensory areas (S1/S2 and AIP) are functionally and causally relevant for the guidance of grasping actions and/or object perception. These experiments will shed new light on sensation-specific (vision vs. touch) and task-specific (action vs. perception) representations of objects of grasp-related brain areas.
X close |
 |